Interviews
Interview: Chemical computing and a circular economy in Brave Green World
July 19, 2021
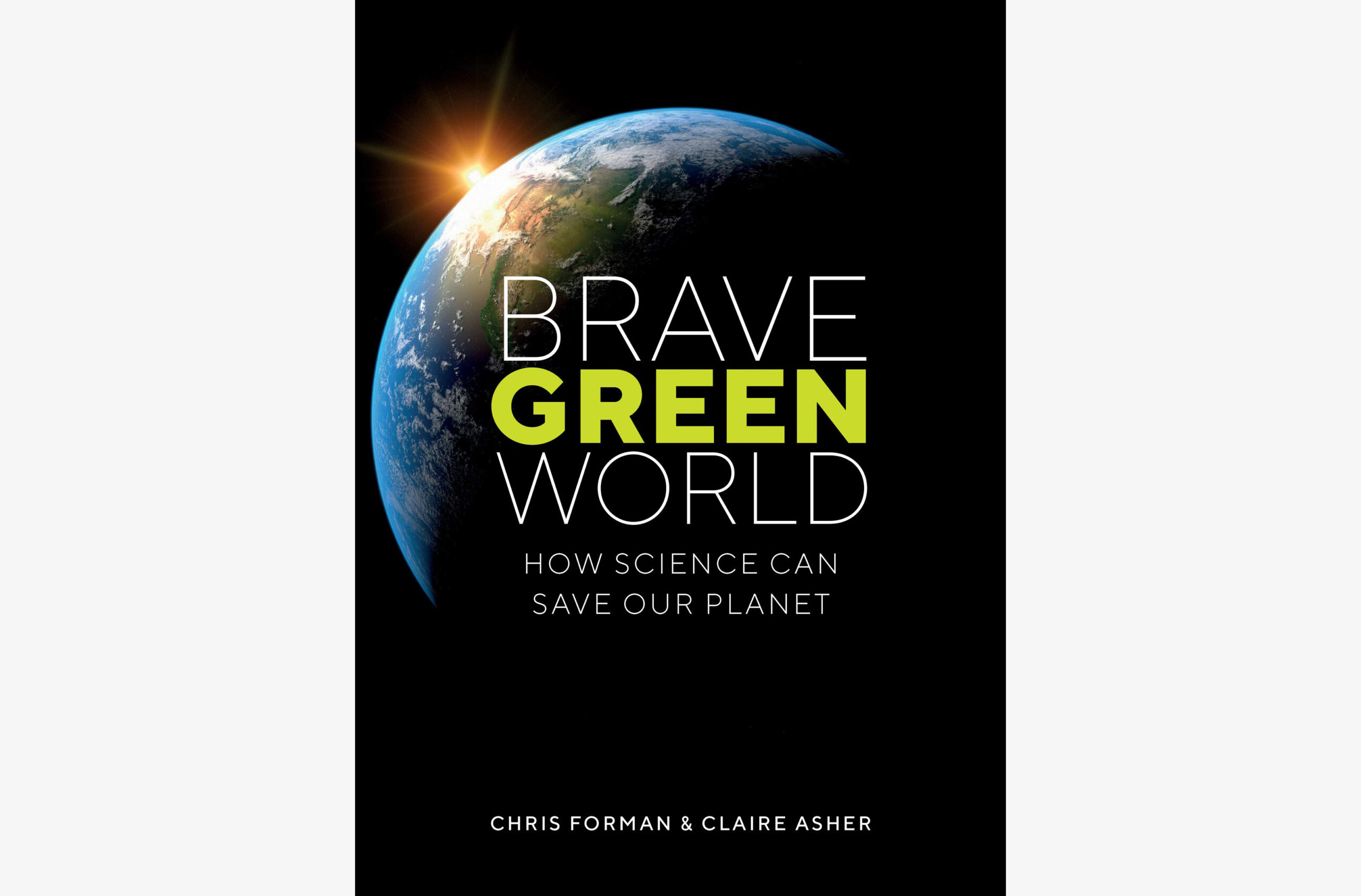
Christopher Forman is the co-author of Brave Green World: How Science Can Save Our Planet, published by The MIT Press. In Brave Green World, Forman and author Claire Asher “offer an unblinkered yet realistic and positive vision of a future in which we can combine biology and manufacturing to solve our central problems of waste and pollution.” Forman also is a research associate in the Gianneschi Group at Northwestern University, whose areas of investigation include biomedicine, nanoscopy, and soft matter and biomaterials.
In the first installment of this two-part interview, Nathan Gianneschi interviews Forman about the concept of a circular economy, and what it could mean for energy and sustainability; how the way we handle waste affects the development of new technologies; and the dizzying possibilities of chemical computing. (Part two is here.)
Gianneschi is the Jacob & Rosaline Cohn Professor of Chemistry, Materials Science & Engineering, and Biomedical Engineering at Northwestern, and the Associate Director of the International Institute for Nanotechnology.
Nathan: What made you want to write a book?
Chris: Back in grad school I wrote a talk called “Can iPods Grow on Trees?” It asked if natural electronics in plants and other biological organisms could inspire a strategy for single molecule electronics, and if we could build something like a smartphone or a computer from the kinds of self-assembly processes we see in nature. I ended up giving a version of this talk at the Cheltenham Science Festival under the title “Natural Nanotechnology”. I met a scientific journalist there named Claire Asher, and we wrote an article together. We realized there was so much material in the subject, there was enough scope for a book.
Nathan: So what’s Brave Green World about?
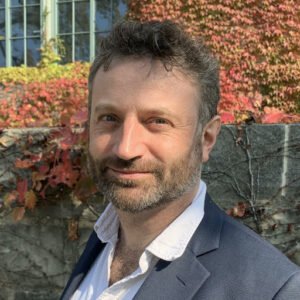
Chris: It’s about technology that exists in the world today, things like 3D printing, additive manufacturing, synthetic biology, AI machine learning, and how we can bring all of those things together to create technology that will enable a circular economy.
In a circular economy, absolutely everything gets recycled and ideally you do that within the energy footprint of the sun. However, while energy considerations are important, I feel this line of reasoning about recycling moves the focus of sustainability away from energy and CO2 emissions and onto the materials that you’re using. How can you build things up in a closed loop system? Is it even possible to manufacture everything you need from recycling? You’ve got to produce entropy. You’ve got to produce waste. How do you create a system that can handle entropy, without drowning in waste?
We argue in the book that looking at the planet as a whole gives a clear answer to that problem. The light coming from the sun mostly leaves Earth again, and in doing so is down-shifted in frequency. For example, blue wavelength light might interact with a molecule causing it to vibrate. As the molecule vibrates it radiates infrared radiation. Roughly the same amount of down-shifted solar energy leaves Earth as there is solar energy arriving, but the number of photons is much higher on the way out. So the entropy of the exiting photons is really high and that change in entropy can be exploited to organize matter on Earth.
Nathan: There’s some energy stored, though, isn’t it? But it’s a very small amount that’s stored in some way, in a plant?
Chris: In a covalent bond. In a plant.
Nathan: But that’s a very, very small percentage.
Chris: I think of it like a capacitor. It charges up and then it hits a steady state where the input equals the output and the entropy change of that radiation is what pays for the organization of all the processes in it, at the molecular scale. And biology is the master of that.
Nathan: That’s the planetary scale.
Chris: Yes. That’s the outline of the jigsaw puzzle. The thermodynamics of the whole of the Earth are well understood. And everything that we want to do must be inside that jigsaw puzzle boundary.
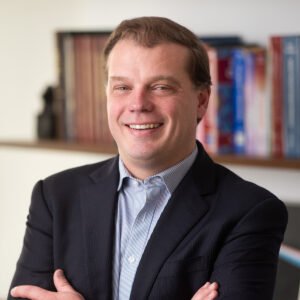
Nathan: We’ll come to the role of nanoscience and the biological tools that are at that scale, and then how that may relate to human technology. But you have a few areas in the book about different kinds of technology. One is synthetic biology. But the first one is additive manufacturing. What’s the importance of that in, not just the nanoscience, but the full scale?
Chris: You’ve got energy, you’ve got matter, and you’ve got information. Those are basically everything in the universe. And what 3D printing achieves is the combination of matter into structures using a stream of information and an appropriate energy source. What does that mean? It means I can build pretty much any object that I want, wherever I am, using the same apparatus. I can use the same infrastructure and add information, without changing the hardware. You can put in a new stream of information and get a new object out. And that’s exactly the way that biology works. Right at the heart of biological systems is the ribosome, and that is essentially a 3D printer.
Nathan: That’s a make-on-demand kind of synthesis machine that the cell uses?
Chris: Yes. The ultimate nanotechnology, in a way.
Nathan: But there are building blocks and things that are consumed by cells, tissues, organisms like us. We were just talking about oxygen transfer. Not everything’s local. So there’s still a need for things to be transmitted around.
Chris: I don’t know exactly what the material constructs of one of these self-grown mobile phones will be. Chances are it needs a bit of every element. And on Earth, all the elements exist in clumped forms, so I feel like we’re going through a phase in the growth of humanity. We’re finding the lumps of stuff and then getting it out, spreading it.
Nathan: So it’s like there’s a capitalization phase. This is a vision for the future, for what do we do when we reach that state where we have enough material distributed.
Chris: Well, one thing is that we shouldn’t export waste because that’s our resource for the future that we’re just handing over to other people. So, you want to get a landfill that’s uniform everywhere.
Nathan: But also the minerals aren’t distributed in rare earth or lithium production, which is a huge problem. And you talk a little bit about batteries. So what’s the role of nanoscience?
Chris: There’s a connection between what happens at the nanoscale and what happens at the planetary scale. We talk about that in the book through the prism of photosynthesis and respiration. There are proteins, and these proteins are nanoscale structures that can handle energy transport. They can catalyze reactions. They can do all kinds of molecular scale things that create the building blocks that you need to add up into larger scale structures. But at the same time, they’re outputting waste into the environment. So in a sense, they’re collectively curating soil, atmosphere, and oceans, which reach a steady state. And all of this happens one molecule at a time across the entire planet, within the global entropy budget from the radiation.
What I find amazing is that biology is the same everywhere. Every cell we’ve ever seen has DNA and proteins. It’s a really successful model that’s spread out around the world.
Nathan: There isn’t another version. At least one that’s survived, that we can detect.
Chris: Right. Maybe in prebiotic evolution there was, but the central dogma paradigm – biology as we know it – is the one that won.
Nathan: So the nanoscale biological assemblies are obviously built, they’re molecular scale and they are similar to these machines, and there’s all kinds of multiple protein interacting-type machines that drive cellular processes. For a lot of what’s been done in the field, supramolecular or self-assembly and nanoscience, biology has been the inspiration. But the synthetic biology component you talk about is leading towards this idea: how do we utilize biological entities to make what we need in a distributed way?
Chris: In terms of complexity, going from the molecular to the cellular is by far the biggest sort of jump. That’s the biggest simplification step. Individual cells are way more complex than sets.
Nathan: Sets of individual nucleic acids?
Chris: Sets of cells. I mean in your body, there’s around 200 different types of cell. But inside a single cell there’s about 10,000 different species of molecule, and so intracellular complexity must be higher than intercellular complexity. You can use synthetic biology to leapfrog up to this point of managing complex sets of molecules. It’s basically a ready-made tool.
Nathan: You said in the book, I think, an $18 billion investment. Is that worldwide?
Chris: Mostly in the U.S. and the U.K., but other countries, private companies. And that was in 2018, so it has probably gone up by now.
Nathan: My understanding of the field is that there’s a lot of research going on in materials, but most of what’s been done in start-up companies has been around generating fuels, small molecules, and the like. It’s mostly been about chemical production.
Chris: Companies like Bolt Threads have been making synthetic silk. There’s another company, Air Protein, that’s synthesizing protein molecules directly from carbon dioxide and water, which is amazing. And there’s other companies that are using fungi as a basis to create synthetic leather. So it’s not just small molecules or pharmaceuticals or fine chemicals. They’re getting into materials.
Nathan: So you would say the tools are coming into place?
Chris: That’s right. And the challenge that I see, once you’ve got these tools and investments and the knowledge, is to pull disparate technologies together to create technology combinations that are incredibly advanced.
Let’s say you assume that we could have these technologies and they work. We’ve got materials, they’re sustainable. There’s a closed loop system. We’ve got the energy to drive it. How then do you connect that stuff together?
That’s what links directly to the research that I’ve been doing for the last three or four years. Like the idea that you could do computation inside chemicals. And this ties directly into the 3D printing idea that you’re putting information into a chemical system. So let’s say, for example, that you could somehow magically inject information into a bunch of chemicals and then the natural processes that take place inside those chemicals are going to morph that information. At the end, you can read out what’s happened. You are then information processing using the natural chemical processes.
The question is, can you program that meaningfully? Can you set that up in such a way that it’s isomorphic – i.e. in one-to-one correspondence – with mathematics? Can I put mathematics inside the chemistry and do the math? If you can do that, you can do exactly what our laptops and our computers do, but in chemicals.
There’s a direct analogy there. Each computer runs Internet browsers, obeys the same rules everywhere, but every Web page can be different and every laptop can use software that can do different things, locally. But they use the same rules.
Can we figure out how to stitch together all of this technology, which is additive manufacturing, all the synthetic biology, then the nanostructure materials, and then program it? The keyword is programming.
Nathan: And program from the level of the molecular components.
Chris: Exactly. How do you put information and algorithms into that chemical picture? Just as I can change the desktop picture on my laptop really easily, because I’ve got this information processing machine, can I do that on a material basis in my house or in a factory? Can I program the matter around me as easily as changing the desktop background? A chemical computer would be the right kind of tool for doing that.
Nathan: One idea was that the chemical computer itself would be capable of doing enough of a complex calculation that it can compute things about the molecules themselves. You need the chemical computer to figure out and enable us to even compute what is going on. That it will give us a better model for future molecular transformation.
Chris: Whereas in a transistor, it can do zeros and ones, basically. If the computing primitive, as they say, was like a protein folding, well, then, that’s got to be a better language for describing protein folding. How do you take advantage of the more complex processes in the chemical systems to enrich the computational primitives that you have available? You can do way more complex calculations with less effort.
Nathan: With the technology for doing that and implementing that computer, is there a scale element? You’ve been doing a lot of work in the realm of, how do you make it? How do you miniaturize it? And then, ultimately, do we know enough about nanoscience and can we build enough nanoscale materials to enable you to do it? Is that where you see a role for nanotechnology?
Chris: Yeah. You can implement the chemical computer at the macro scale with large bulk stuff but you don’t get a computing advantage from that. You get the computing advantage when you shrink it down to the size of cells.
Nathan: Why do you get an advantage at the nanoscale?
Chris: For a start, you can just fit in more computing elements into a volume.
Nathan: You don’t have to fill a warehouse with chemicals.
Chris: They’re essentially bulk systems. They’re not two dimensional systems, like chips are essentially two dimensional with many layers, but they’re still two dimensional.
Nathan: There’s also the chemical engineering part of it. There’s practical things about the diffusion rates for example. If you make it very small, you can overcome some of those barriers.
Chris: Yes. The amount of heat it generates for example.
Nathan: Because otherwise you have the classical cooling system issues and beyond.
Chris: Like our bodies work. Trillions of cells operating in parallel and maintaining a steady heat output. Parallel computing is the way forward, in terms of power of computing.
Nathan: So, the miniaturization. We’ve looked at droplet arrays around this idea of different types of effects that can be observed within confined environments and droplets. There’s a lot of questions around reaction confinement, around reactivity and interfaces. And this is all kind of built into these systems?
Chris: Yes. The surface area is much higher, so the interfacing complexity is much higher. Think about the brain with the staggering number of connections that you have there.
But where I’m really excited with chemical computing – that’s brand new and completely different from regular computing – at the end of your chemical computation, you’ve configured a bunch of molecules into a particular state. So not only have you done a computation, but you’ve also made something.
I’m really excited by that prospect. That’s something that microchips just cannot do. So it’s worth taking a hit in the computational performance if you’re going to make something that’s useful. Because Turing machines can run any algorithm and do any computation, can we get all of the cool computational science into our manufacturing?
Nathan: And that is a concept you outlined in the book.
Chris: Yes. We were inspired by the Internet. At the end of the book we bring all these ideas back together and ask what networks of chemical computers look like. We call it “The Synthernet” and it could link all this wonderful technology like 3D printing and synthetic biology into a platform technology that forms the basis for a circular economy. We spend some time having fun imagineering about what the future might look like with this kind of technology. That’s the prize for struggling through the whole book!
Brave Green World: How Science Can Save Our Planet is published by The MIT Press.